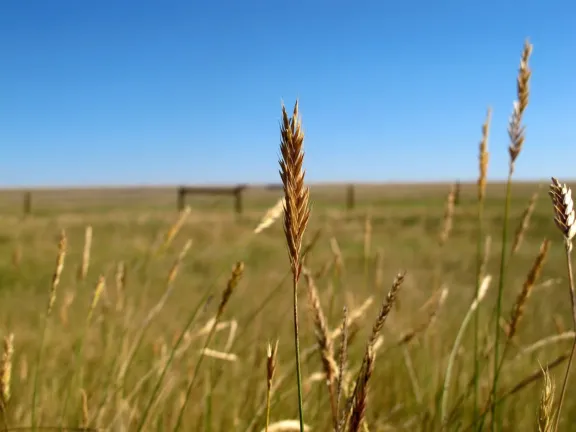
The Science of Food: The Safe GMO and the Un-boiled Egg
GMO containment
Humans have been developing genetically-modified organisms for millennia through the process of artificial selection—human-directed selective breeding—of plants and animals for specific traits. For example, broccoli, cauliflower, cabbage and kale are all descended from the wild mustard plant, and there are now hundreds if not thousands of dog breeds.
Laboratory-based genetic modification was first developed the 1970s, and applications toward improving the quality of human life were soon to follow. Calgene Inc.—a biotech company based in Davis, California—brought the first FDA-approved GMO crop to the commercial market in 1994.
Many years and commercialized GMOs later, a divided public continues to debate the safety and ethics of this technology fiercely, mostly on the grounds of myths and misunderstandings. One worry that is grounded in fact, however, is that GMO plants will cross-pollinate with neighboring wild plants, passing the edited gene throughout an ecological system. To the disappointment of some in the science community, the science educator Bill Nye said in November 2014 that “although you can know what happens to any individual species that you modify, you cannot be certain what will happen to the ecosystem.” While all other anti-GMO arguments are easily debunked, this one has been difficult to address.
Now, two groups of scientists have made significant progress toward overcoming this obstacle. Two publications in Nature, published online on Jan. 21, both described synthetic safety switches to ensure GMO control and containment. The groups, led by Farren Isaacs from Yale University and George Church from Harvard University, semi-independently engineered E. coli bacteria such that their altered biology makes it very nearly impossible for them or their DNA to escape into the wild.
Let me back up. I say semi-independently, because both publications were based on a previous joint 2013 publication between these two groups that developed the world’s first “genomically recoded organism.” This remarkable strain of E. coli bacteria carries an altered genetic code – which is otherwise conserved among all living organisms.
Cells make proteins based on RNA instructions. Each three-nucleotide combination calls for a particular amino acid. Three of these sequences, however, have no matching tRNA molecule to bring the correct amino acid and therefore act as a stop command. To make the genomically recoded organism, the scientists meticulously removed every instance of one of these stop sequences, TAG, from E. coli strain’s entire genome, replacing it with a different stop sequence. They then reintroduced the TAG sequence into strategic sites in the bacterial genome along with a matching engineered tRNA.
You can already see where they were headed with this painstaking engineering project: toward organisms with a completely alien form of basic molecular biology that makes them a) incompatible with all normal life on earth, and b) irreversibly dependent on synthetic biological building blocks not found anywhere in nature.
This proof of concept is where the 2013 paper stopped, but the logical next step was clearly to give the synthetic tRNA a synthetic amino acid match. And that is exactly what each group has now done.
The two groups used different approaches to decide where to insert the TAG codons and also different screens for potential synthetic amino acids. The Isaacs group screened through 22 different reintroductions and various synthetic amino acids and identified combinations that did not disrupt normal protein function. Church’s group screened 6 different reintroductions, at times mutating flanking amino acids in order to facilitate the process. Interestingly, both groups found success with different synthetic amino acids similar to phenylalanine.
This strategy of containment is impressive in both its elegance and thoroughness. Church’s group wrote that “Effective biocontainment strategies must protect against three possible escape mechanisms: mutagenic drift, environmental supplementation and horizontal gene transfer.” While a GMO crop incorporating this synthetic safety switch—which fulfills all three criteria—is years away, the path there has just become much clearer.
Your move, Bill Nye.
Sources: Nature, Nature News, Harvard News, N.Y. Times, Popular Science, Discover Magazine
Un-boiling an egg
Science news was abuzz last week when UC Irvine released a press release that some of its scientists had figured out how to un-boil an egg—in science terms, to re-fold aggregates of denatured proteins.
The work was a collaboration between Gregory Weiss’ group at UC Irvine and Colin Raston’s group at Flinder University in Australia and was published in ChemBioChem on Jan. 23. The scientists used boiled egg whites—which are 12 percent protein—to demonstrate the power of their technique. They began by boiling egg whites at 90 degrees Celsius for 20 minutes (ensuring that they were so hardboiled that a “Top Chef” judge would spit them out with indignation with the words “These are hammered!”).
They dissolved the hardboiled egg whites in urea—a major component of urine, most news sources were quick to note—to re-solubilize the protein aggregates. They then spun them at room temperature at high speeds in a vortex fluid device—a narrow, glass cylinder that spins rapidly on its axis at a 45-degree angle. The shear stress exerted on the liquid in this device appeared to be enough to coax apart protein aggregates and allow them to re-fold into their natural conformations. This was demonstrated by the rescue of normal lysozyme enzymatic activity and normal caveolin-1 interaction with a protein-binding partner. The protocol had to be tweaked to optimize refolding of a protein kinase A, a bulkier protein, and the authors noted that individual optimization would likely be necessary for others as well.
This process could have major implications for industries that manufacture proteins in bulk—notably, the pharmaceutical and food industries—because these struggle with protein misfolding in yeast and E. coli protein overexpression platforms. Existing strategies to recover misfolded proteins take multiple days. “The new process takes minutes,” Weiss noted. “It speeds things up by a factor of thousands.”
Sources: ChemBioChem, UCI News, Popular Science, IFLScience