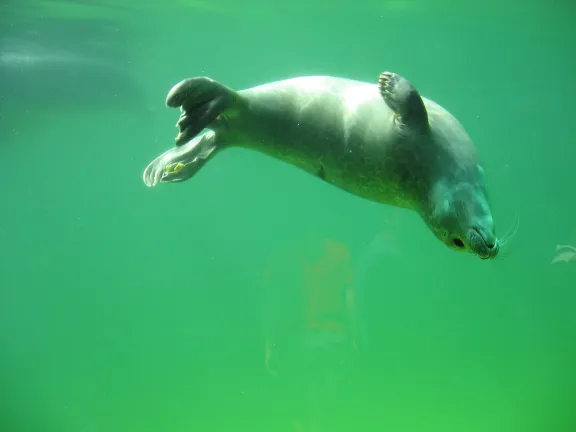
Biologically-Inspired Engineering
Seal-inspired underwater motion sensors
The past few years have seen an increase in robotics inspired by the natural world. These robots are designed to look and move like animals, from flying like a bird to running and jumping like a cheetah. Aside from being intriguing engineering challenges, these types of robotics could also be used as a substitute for humans in dangerous or difficult situations.
For instance, some of these new kinds of robots have competed in the U.S. Department of Defense’s DARPA (Defense Advanced Research Projects Agency) Robotics Challenge, which was launched after the 2011 Fukushima nuclear crisis in Japan and was completed this June: “The goal was to accelerate progress in robotics and hasten the day when robots have sufficient dexterity and robustness to enter areas too dangerous for humans and mitigate the impacts of natural or man-made disasters.”
While some high-profile engineers, including Elon Musk and Bill Gates, are vocally against the hasty development of artificial intelligence, human-controlled robotic drones that harness the diverse movement patterns created by nature would be powerful tools in many situations.
Underwater robotics faces a whole new set of challenges, from placing a lot of functioning electronics underwater to developing good underwater sensors. Professor Michael Triantafyllou’s and his PhD student Heather Beem in the MIT Department of Mechanical Engineering approached this issue by turning to the natural world. Triantafyllou and Beem studied harbor seal whiskers and created their own artificial “whisker” sensor, published this month in Journal of Fluid Mechanics, that could help underwater robotic vehicles sense and track moving objects including animals and pollutants.
These MIT engineers started with the observation that harbor seals have an “outstanding ability to detect and track hydrodynamic signatures left by swimming animals and moving objects.” Even when blindfolded, the seals can follow the tracks of their prey up to 30 seconds after they swam by. The “tracks” left by objects moving through water are in the form of vortex wakes - turbulent whirlpools left behind like dropped breadcrumbs - and harbor seal whiskers have evolved to be particularly attuned to such fluid dynamics.
The whiskers have an elliptical cross-section of sinusoidally-varying width, which gives them a wavy side-view. Computer simulations and physical tests of an artificial whisker both showed that while it remains still in the context of the seal’s own swimming motion, this whisker shape vibrates in response to other nearby movements.
The authors described this vibration as a “slaloming trajectory” - like the motion of a downhill skier zigzagging around slalom gates - which synchronizes to the vortex wakes left behind by the passing object.
Thus, harbor seal whiskers align themselves to the path left behind by a passing fish, and the larger the fish, the easier it is for the whisker to lead the seal to its next meal. Thanks to the increasing demands of 21st century robotics, we now understand a little more about seal anatomy and physiology. Furthermore, because “slaloming” has also been observed in swimming trout, the authors of this study speculate that it is a fundamental mechanism of extracting energy from flowing water that can be applied to harnessing energy from water or air.
Sources: Journal of Fluid Mechanics, MIT News, DARPA Robotics Challenge
Skin-inspired prosthetic tactile sensors
Professor Zhenan Bao and her group in Stanford University’s Department of Chemical Engineering have been working for ten years to create an artificial material that can mimic the skin’s sense of touch, and this month in Science, they’ve published their successful creation of a plastic skin-like material that can detect and distinguish forces of pressure.
The “Digital Tactile System,” or “DiTact,” is a flexible material made up of pressure sensors, electronic oscillators, and live mouse cortical neurons. As more force is applied to the material, the pressure sensors stimulate increasing frequency of the oscillators’ electric signal, which turn on an LED light source, stimulating an action potential in channelrhodopsin-expressing light-activated neurons. This novel bioelectric material responds to mechanical forces and translates them into biological signals, utilizing optogenetic neurons as proof of principle but ultimately aiming to link prosthetics to human neurons.
While this new material is an impressive feat of engineering and a breakthrough for the field of smart prosthetics, it is just the first step in creating true artificial skin. The DiTact material can detect and send signals of pressure strength to neurons, but the complete sense of touch is a combination of signals from mechanoreceptors (pressure, vibrations, and texture), thermoreceptors (temperature), pain receptors, and proprioreceptors (motion and position of one’s own body).
Professor Bao’s group intends to tackle some of these aspects of touch next, including texture and temperature. "We have a lot of work to take this from experimental to practical applications… But after spending many years in this work, I now see a clear path where we can take our artificial skin."
Sources: Science, Scientific American, Stanford News